A quick note to promote Coursera, an aggregator of online classes–if you’re planning to take Physiology anytime soon, I highly recommend taking it here first as a primer, especially if you have no familiarity with the material. The Duke University curriculum and instructors are excellent–and free! You should also consider it if you’ve already taken physiology but feel like you need to brush-up before taking the NLN PAX.
Now on to the nervous system–our nervous system, like the circulatory system, engages every part of our body. But instead of veins, arteries and capillaries, it’s comprised of axons, dendrites and glia, conducting electricity. Working in conjunction with the muscular system, it allows us to move and breathe. And because the heart is a muscle, it keeps the engine of the circulatory system humming. It helps to keep our vitals systems in homeostasis by communicating dangerous rises or drops in blood pressure, heart rate, pH, O2 and CO2 levels and sometimes releases neurotransmitters into the blood to move these systems back to setpoint. Lastly, it communicates a variety of sensory messages to the brain.
So how does all this communication happen? There are two main components: the electrical signal, and the network on which it travels.
The Electrical Signal
The fluid inside and surrounding our cells (intracellular fluid, ICF, and extracellular fluid, ECF) is filled with chemical elements—mostly sodium (Na), Potassium (K), Calcium (Ca), and Chloride (Cl). If an element has more or fewer electrons than protons, it has an electrical charge and is called an ion. The charge is measured, + or -, in millivolts (mV). Remember that.
A plasma membrane, the protective wrapper of the cell, separates the ICF and ECF. The mix of ions in the ICF and ECF determines its voltage, and the difference in voltage between the ICF and ECF determines the membrane potential for that cell. For example, at rest, most neurons have lots of K+ in the ICF and lots of Na+ in the ECF. The difference in voltage is about -70 mV, and this is the resting membrane potential (RMP) for the cells of our nervous system, aka neurons.
When a stimulus occurs–the smell of pancakes, a sting on your leg, even a slight breeze on the hairs of your forearm–voltage-gated Na+ channels on the plasma membrane open and Na+ rushes into the cell. This changes the difference in voltage between the ECF and the ICF and the membrane potential rises–depolarization of the neuron begins. Remember, it starts at -70 mV, the RMP. If the stimulus is strong enough, and enough Na+ moves into the cell, the membrane potential rises to +30 mV, and an action potential, or electrical signal is fired. The action potential then travels the length of the axon to your brain, where the stimulus is registered and another signal is sent from the brain to your muscles–you decide to eat pancakes, move your leg, or put on a jacket.
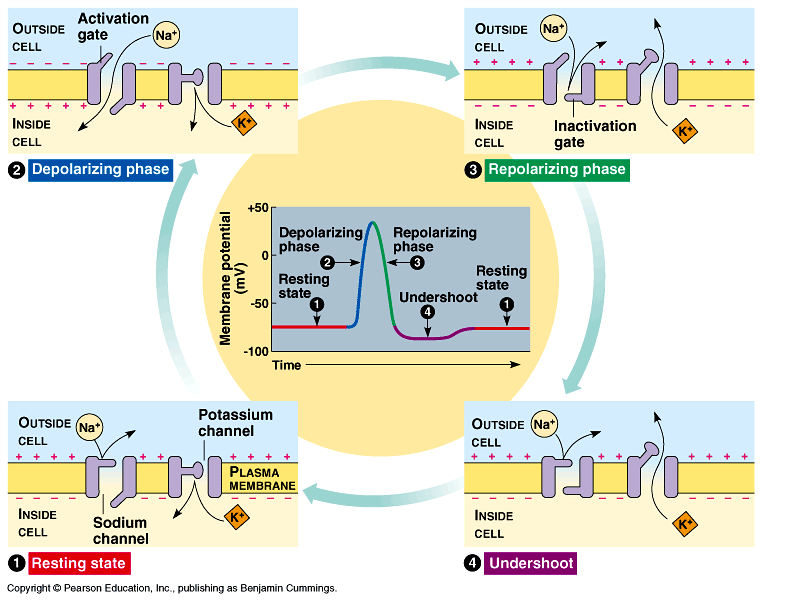
Once the membrane potential reaches +30 mV and the action potential fires, the Na+ channels shut and the cell repolarizes. If the stimulus continues, another action potential is fired. Of course this all happens in femto seconds and is happening in more than one neuron–your fingertips have as many as 100 touch receptors per cm2.
An example of a medication that tweaks our nervous system, as many of our medications do, is lidocaine, which binds and inhibits voltage-gated Na+ channels. Liocaine is what the dentist injects into your gums before drilling your teeth. By inhibiting the voltage-gated ion channels of the neurons in your gums, there is no Na+ entering the cell, no cell depolarization, no action potential fired–no stimulus of the pain/nociceptors, and no pain felt.
That’s a lot of info–welcome to #nurselife! And that’s probably enough info for now. I’ll cover the network that all these signals travel upon next time–the information highway of our bodies! 😉